In Mary Shelley´s groundbreaking science fiction novel, Frankenstein, Professor Victor creates a grotesque monster by stitching together human body parts.
Two hundred years later, Shelley’s idea has (in a certain way) become a reality: hundreds of thousands of organs are transplanted from one person’s body into another, saving lives every year. So… what is the next step? Have you ever wondered how close are scientists to being able to grow working organs in a dish?
Today, model organs are a reality, and they’re called organs-on-chip. They are engineered devices that mimic the complex structure of organs such as lungs, guts, and kidneys. The ability to grow these “mini-organs” has taken off in the last few years with the latest advances in bioengineering that uses technology designed to manufacture computer microchips.
While these mini-organs are not quite ready for transplanting into patients, they play important roles in helping scientists understand how the human body works better and to find new cures for diseases. Scientists have been studying human cells grown in culture dishes for decades.
However, you may be thinking that the stiff, flat surface of culture flasks doesn’t bear much resemblance to the human body. You’re right – the lab is missing the enormous complexity of the human body, where cells grow in 3-dimensional tissue structures and communicate with each other to constitute functional organs. In fact, cells kept in the lab for too long change their original behavior, and can potentially give misleading results. In addition, a complex phenomenon such as blood flow, muscle movements in the guts or air pressure in the lungs can’t be monitored in traditional lab cultures.
That’s where 3D modeling and organs-on-chip come in. At the Center for Infectious Disease Research, we are collaborating with bioengineers at the University of Washington to develop a 3D “brain microvessel model” to study cerebral malaria.
Cerebral malaria is one of the most life-threatening complications of malaria. It is responsible for most of the 400,000 deaths that malaria causes every year. It’s caused by malaria-infected red blood cells sticking to the brain capillaries. These sticky parasites release toxins that can cause brain swelling and break the blood-brain barrier, a network of specialized blood vessels in the brain that is 500 times stronger than capillaries in the rest of the body. The blood-brain barrier shields the brain from toxic chemicals in the blood and maintains the perfect environment for neuronal function.
During a cerebral malaria episode, patients – usually children under five years old – lose consciousness, falling into a coma. Some children develop brain swelling due to the breakdown of the blood-brain barrier. The swelling can sometimes be so massive that the brain is forced out through the bottom of the skull, pressing on the brain stem (at the top of the spinal cord) and compressing the area that controls breathing.
The only option is hoping antimalarial drugs can clear their body of parasites quickly enough for the patient to survive the swelling of their brain – a treatment with a 20% failure rate. If a drug could reverse the brain swelling, the antimalarial drugs would have more time to work, increasing the patient’s chances of survival.
That drug doesn’t exist.
Understanding just how malaria parasites damage the blood-brain barrier can lead to the design of new drugs that specifically reverse the swelling caused by the parasite. As the blood-brain barrier has a complex 3-dimensional structure that is quite difficult to recreate on the flat plane of a culture flask, the Smith lab is trying to recreate what happens in the brain of cerebral malaria patients using the most realistic scenario.
The new 3D microvessel model offers a unique opportunity. The devices, no bigger than the size of a dime, are composed of a scaffold made with collagen, the main structural protein in animal tissues. The collagen scaffold is crossed by tiny tube-shaped holes, simulating the space of a capillary blood vessel. In the organ-on-chip, capillary brain cells create a barrier between the blood (simulated by the open channel) and the tissue (simulated by the collagen)
In the microvessel, we can flow malaria-infected red blood cells through the open channel to see how parasites open the barrier, and test drugs and strategies to reduce brain swelling.
Even though we are still relatively far away from growing completely functional organs in our lab, the technology to model the function of organs is a priceless tool to understand diseases.
Source & Credit @ Center for Infectious Disease Research and was written by Maria Bernabeu.
Thursday, 15 March 2018
Fact or Fiction? Growing Body Parts in a Lab
Subscribe to:
Post Comments (Atom)
Daily Weapon to Combat Climate Change
The continuous exploration of Mother Earth is drifting the balance and threatening livelihood daily. This isn’t just making it difficult to...
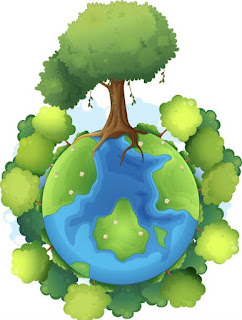
No comments:
Post a Comment